By Sjoerd Willem Duiker
Figure 1. Pictures taken on July 9, 1 month after planting. On the left is corn in compacted area showing lower stature and purple coloring on the leaves. On the right is corn in uncompacted area showing taller stature, deeper green color and no purple coloring. Corn on the left tested low (0.18% P) while that at the right tested normal (0.31% P).
This spring we compacted three strips in a field for our diagnostic clinic with a 30 ton loaded tri-axle truck with road tires. Axle load was 6-12-12 tons on front, first and second rear axles, respectively. The tires were inflated to 105 psi. These trucks are frequently used to haul corn silage or grain and are even used to haul manure. We caused the compaction on May 2nd by driving back-and-forth on the field to cover 100% of the ‘compaction strip’ one time. The soil was quite moist – about 30% at the surface, increasing to up to 45% at 12 inches deep. It was definitely in the ‘plastic condition’. However, deep ruts were not created. The soil was chisel plowed and harrowed after compaction and planted to corn in early June. Soil compaction research shows that above 10 ton axle load subsoil compaction threat becomes high, while high surface stress due to small footprint of road tires inflated to high pressure results in severe surface compaction.
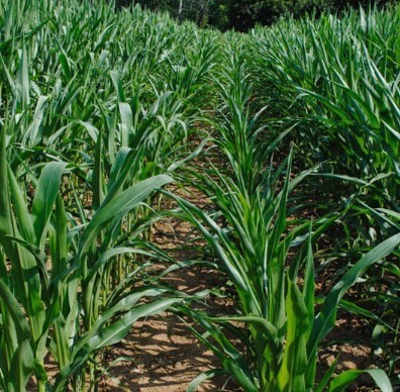
Figure 2. Corn in compacted area showing drought stress and short stature due to the effects of soil compaction.
The results of compaction on the soil and crop was quite striking after the second leaf stage of corn (Figure 1). It seemed chisel plowing loosened the surface soil, facilitating early growth, but once the roots hit the compacted layer underneath, growth of the corn started to become severely limited. We noticed that the penetration resistance increased dramatically at about 9 inches depth and root growth was severely restricted at that level, compared with the uncompacted area of the field. The soil below that depth had a massive structure while it had ‘blocky’ structure in the uncompacted area. Surface soil structure was much more crumbly in the non-compacted areas while it was more cloddy in the compacted areas. This showed that the larger pore spaces had been lost and the soil structure destroyed by the compaction. The purpling of the leaves was a sign of phosphorus deficiency. Phosphorus is quite immobile in the soil so the roots ‘have to go get it’. The restricted root systems caused the uptake of phosphorus to be limited. We ran a tissue test and the phosphorus levels were ‘low’ (0.18%) in the compacted areas, but ‘sufficient’ (0.31%) in the uncompacted areas, confirming our field observations. We also saw some signs of potassium deficiency, another relatively immobile element in the soil. Nitrogen deficiency was not that evident. Nitrogen is a highly mobile element and as long as water can move from the soil solution to the roots it will carry nitrogen with it. However, nitrogen deficiency can also be common in compacted soils in lower parts of a field due to restricted drainage that leads to saturated conditions resulting in nitrogen loss due to denitrification.
Last week we took another look at the corn and noticed that it was a lot shorter and showing drought stress in the compacted strips (Figure 2). The interesting observation was that the soil between the rows was 10% higher in the compacted than in the non-compacted areas, showing that the moisture was there but there were no roots to take it up in the compacted areas. We noticed more severe effects of compaction where soil showed a red shine signifying higher clay content. Clay particles are plate-shaped and when water surrounds them they tend to slide together and pack real tight resulting in more severe compaction potential. Our limestone soils naturally have a silt loam surface soil but in these areas the topsoil had been lost due to erosion. These observations showed us how important it is to reduce compaction by first of all avoiding it, and, if needed, remediating it. In a future issue of Field Crop News we will talk more about how to achieve that. For now, I hope you will remember the potential future effects of hauling corn silage with triaxle trucks mounted with road tires.Last week we took another look at the corn and noticed that it was a lot shorter and showing drought stress in the compacted strips (Figure 2). The interesting observation was that the soil between the rows was 10% higher in the compacted than in the non-compacted areas, showing that the moisture was there but there were no roots to take it up in the compacted areas. We noticed more severe effects of compaction where soil showed a red shine signifying higher clay content. Clay particles are plate-shaped and when water surrounds them they tend to slide together and pack real tight resulting in more severe compaction potential. Our limestone soils naturally have a silt loam surface soil but in these areas the topsoil had been lost due to erosion. These observations showed us how important it is to reduce compaction by first of all avoiding it, and, if needed, remediating it. In a future issue of Field Crop News we will talk more about how to achieve that. For now, I hope you will remember the potential future effects of hauling corn silage with triaxle trucks mounted with road tires.